Electric Vehicle Battery Usage
Electric vehicle battery usage encompasses energy storage, power management, and efficiency in EVs. These batteries power the vehicle’s motor, enabling emission-free driving. They are critical for range, charging speed, and overall vehicle performance. Innovations in battery technology focus on extending lifespan, reducing costs, and enhancing energy density for improved electric mobility.
Electric Vehicle Battery Usages: A Comprehensive Guide
The rise of electric vehicles (EVs) marks a significant shift in the automotive industry, driven by the need for sustainable and eco-friendly transportation solutions. Central to the operation of electric vehicles is the battery, a crucial component that not only powers the vehicle but also influences its performance, range, and overall efficiency. This blog post delves into the various aspects of electric vehicle battery usage, exploring the types of batteries used, their life cycles, charging practices, technological advancements, and the future of EV batteries.
Electric Vehicle Battery Usage
1. Types of Batteries Used in Electric Vehicles
Electric vehicles primarily use two types of batteries: Lithium-ion (Li-ion) batteries and Nickel-Metal Hydride (NiMH) batteries. Each of these battery types has distinct characteristics that make them suitable for different applications.
Lithium-ion Batteries
Lithium-ion batteries are the most common type used in modern electric vehicles. They are known for their high energy density, which allows them to store more energy in a compact space, leading to longer driving ranges for EVs. Additionally, Li-ion batteries have a relatively long lifespan and are lightweight, which contributes to the overall efficiency of the vehicle.
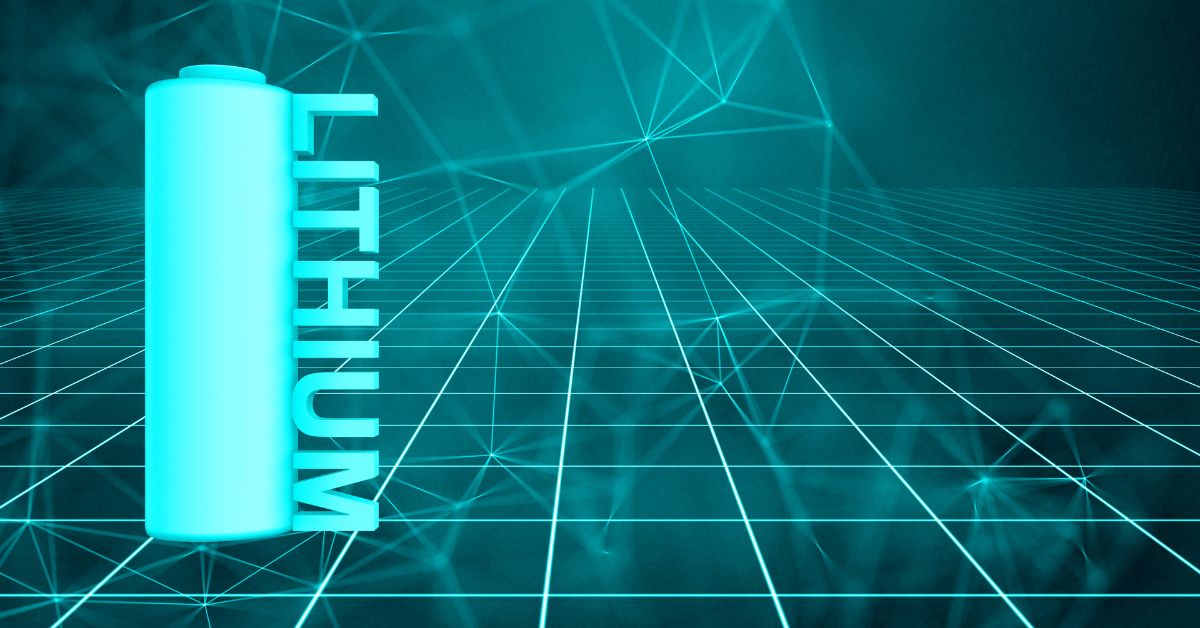
Advantages:
- High energy density
- Long cycle life
- Lightweight
- Fast charging capabilities
Disadvantages:
- High cost
- Potential for thermal runaway if not properly managed
- Environmental concerns related to the extraction of lithium and other raw materials
Nickel-Metal Hydride Batteries
Nickel-metal hydride batteries were commonly used in early hybrid vehicles but have since been largely replaced by Lithium-ion batteries in fully electric vehicles. However, they are still used in some hybrid electric vehicles (HEVs) due to their reliability and safety.
Advantages:
- Proven track record in hybrid vehicles
- Robust and durable
- Less prone to overheating
Disadvantages:
- Lower energy density compared to Li-ion batteries
- Heavier and bulkier
- Slower charging times
2. Battery Life Cycle and Degradation
Understanding the life cycle of an electric vehicle battery is essential for consumers and manufacturers alike. The performance and lifespan of a battery are influenced by several factors, including usage patterns, charging habits, and environmental conditions.

Battery Life Cycle
The life cycle of an EV battery is typically measured in charge-discharge cycles. A cycle refers to the process of charging a battery from 0% to 100% and then discharging it back to 0%. The number of cycles a battery can undergo before its capacity significantly degrades is a key determinant of its overall lifespan.
Most Lithium-ion batteries used in electric vehicles are designed to last between 1,000 to 1,500 charge cycles, which translates to approximately 8 to 15 years of use, depending on the driving habits and maintenance.
Factors Influencing Battery Degradation
Several factors can accelerate the degradation of an electric vehicle battery, reducing its lifespan:
Temperature:
- Extreme temperatures, both hot and cold, can negatively impact battery life. High temperatures can cause the battery to overheat, leading to thermal degradation, while low temperatures can reduce the battery’s efficiency and capacity.
Charging Practices:
- Frequent fast charging can lead to increased wear and tear on the battery, as it generates more heat and stress compared to slower, regular charging. Additionally, regularly charging the battery to 100% and discharging it to 0% can accelerate degradation. It is recommended to keep the battery between 20% and 80% charge for optimal longevity.
Depth of Discharge:
- The depth of discharge (DoD) refers to how much of the battery’s capacity is used before it is recharged. A lower DoD (partial discharging) generally results in a longer battery life, while deep discharging (using the battery until it is almost empty) can shorten its lifespan.
Cycling Frequency:
- The more frequently the battery is cycled (charged and discharged), the faster it will degrade. For this reason, vehicles with high daily mileage or those subjected to heavy loads may experience faster battery degradation.
3. Charging Practices and Their Impact on Battery Health
Charging is a critical aspect of electric vehicle battery usage, and the way a battery is charged can significantly influence its performance and longevity. This section covers the various charging methods and their effects on battery health.

Types of Charging
Electric vehicles can be charged using different methods, each with its advantages and drawbacks:
Level 1 Charging (Standard Outlet):
- Level 1 charging involves plugging the EV into a standard 120-volt household outlet. This method is the slowest, typically adding 3-5 miles of range per hour of charging. While it is convenient, especially for overnight charging, it is not suitable for drivers who need to recharge quickly.
Level 2 Charging (Home or Public Chargers):
- Level 2 charging uses a 240-volt outlet, similar to what is used for large home appliances like dryers. This method is faster, adding about 20-30 miles of range per hour. Level 2 chargers are commonly found in homes, workplaces, and public charging stations, making them a popular choice for daily charging.
DC Fast Charging:
- DC fast charging (Level 3) is the quickest method, capable of charging an EV battery to 80% in as little as 30 minutes. This method is ideal for long trips or when a quick recharge is needed. However, frequent use of DC fast charging can lead to increased battery wear due to the higher temperatures and stress involved.
Best Practices for Charging
To maximize battery life and maintain optimal performance, EV owners should adopt the following charging practices:
Avoid Frequent Fast Charging:
- While fast charging is convenient, it should be used sparingly. Relying primarily on Level 1 or Level 2 charging can help preserve battery health.
Maintain a Moderate State of Charge:
- Keeping the battery charge level between 20% and 80% can extend its lifespan. Avoid letting the battery charge drop too low or charging it to full capacity too often.
Charge in a Temperature-Controlled Environment:
- Charging the battery in a temperature-controlled environment can prevent thermal degradation. Avoid charging the vehicle in extremely hot or cold conditions whenever possible.
Use Regenerative Braking Wisely:
- Regenerative braking, which converts kinetic energy back into stored energy in the battery, can help extend the range of an EV. However, aggressive use of regenerative braking can generate heat and stress the battery, so it should be used judiciously.
4. Technological Advancements in EV Batteries
The ongoing research and development in battery technology are driving the evolution of electric vehicles, leading to more efficient, longer-lasting, and safer batteries. This section highlights some of the most promising advancements in EV battery technology.
Solid-State Batteries
Solid-state batteries are considered the next big leap in battery technology. Unlike traditional Lithium-ion batteries that use liquid electrolytes, solid-state batteries use a solid electrolyte, which offers several advantages:
Higher Energy Density:
- Solid-state batteries can store more energy in a smaller space, potentially doubling the range of electric vehicles.
Improved Safety:
- The solid electrolyte is less prone to overheating and catching fire, making solid-state batteries safer than their liquid counterparts.
Faster Charging:
- Solid-state batteries can charge more quickly and handle higher currents without degrading as rapidly, making them ideal for fast-charging applications.
Longer Lifespan:
- Due to their increased stability, solid-state batteries are expected to last longer than traditional Li-ion batteries, reducing the need for frequent replacements.
Battery Recycling and Second-Life Applications
As the adoption of electric vehicles grows, so does the need for effective battery recycling and second-life applications. These initiatives are crucial for minimizing environmental impact and maximizing the value of EV batteries.
Recycling:
- Recycling technologies are being developed to recover valuable materials such as lithium, cobalt, and nickel from used batteries. These materials can then be reused in the production of new batteries, reducing the demand for raw material extraction and lowering the environmental footprint of EVs.
Second-Life Applications:
- Even after an EV battery has reached the end of its useful life in a vehicle, it can still have up to 70-80% of its capacity remaining. These batteries can be repurposed for less demanding applications, such as energy storage for homes and businesses, extending their overall lifespan and utility.
Wireless Charging
Wireless charging technology is an emerging area of interest in the EV industry. This technology allows electric vehicles to be charged without the need for physical connectors, offering greater convenience and the potential for more widespread adoption.
Inductive Charging Pads:
- Inductive charging pads embedded in the ground can charge an EV parked over them. This technology is already being tested in some public spaces and could become more common in the future.
Dynamic Wireless Charging:
- Dynamic wireless charging involves embedding charging infrastructure in roadways, allowing EVs to charge while driving. This could significantly reduce the need for frequent charging stops, especially on long journeys.
5. Environmental and Economic Impact of EV Batteries
The transition to electric vehicles (EVs) offers promising environmental benefits, such as reduced greenhouse gas emissions and decreased reliance on fossil fuels. However, the production, usage, and disposal of EV batteries have their own environmental and economic implications that need to be carefully considered.
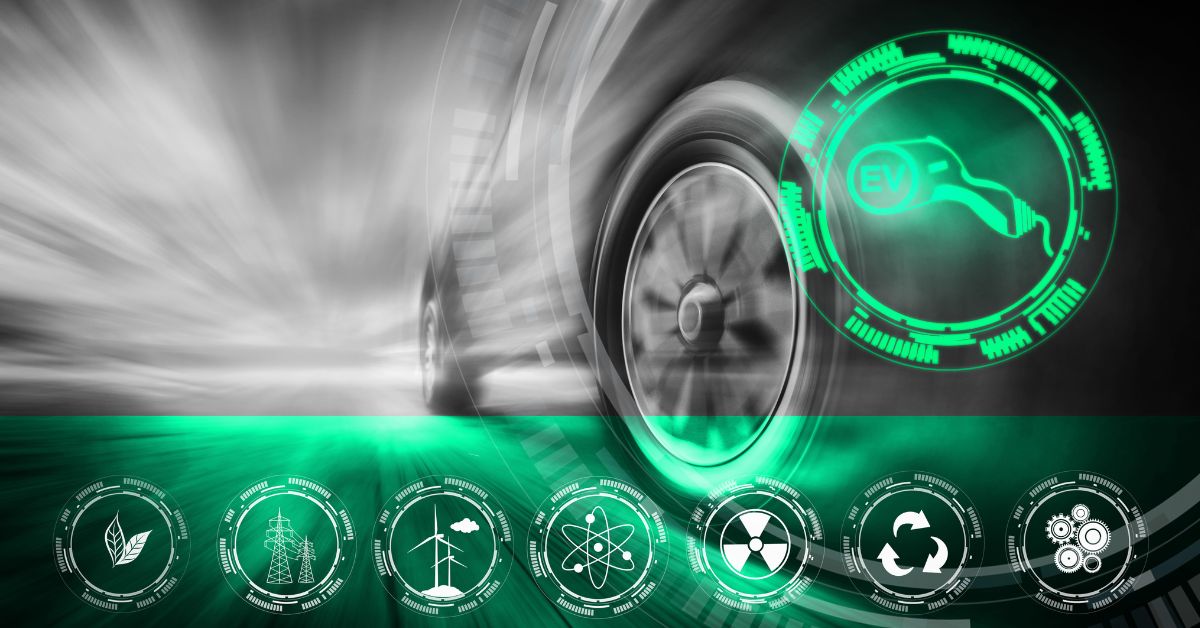
Environmental Impact
Raw Material Extraction:
- Mining and Resource Depletion: EV batteries, particularly Lithium-ion batteries, rely on critical raw materials such as lithium, cobalt, nickel, and graphite. Extracting these materials involves intensive mining operations, often in environmentally sensitive regions. Mining can lead to habitat destruction, soil erosion, and contamination of water resources. Moreover, the extraction process is energy-intensive and contributes to carbon emissions.
- Water Usage and Pollution: Lithium extraction, in particular, is water-intensive, especially in arid regions like the lithium triangle in South America. The process of extracting lithium from brine requires vast amounts of water, leading to the depletion of local water resources and impacting local communities and ecosystems. Additionally, the chemicals used in mining and extraction can pollute surrounding water bodies, harming aquatic life and contaminating drinking water sources.
Manufacturing Process:
- Energy Consumption and Emissions: The production of EV batteries is an energy-intensive process, and the energy mix used in manufacturing can significantly affect the overall carbon footprint of the battery. If the electricity used comes from fossil fuels, the carbon emissions associated with battery production can be substantial. However, as renewable energy sources become more prevalent, the carbon footprint of battery manufacturing is expected to decrease.
- Toxic Waste and Chemical Management: The production process involves the use of various chemicals, some of which are hazardous. Improper handling or disposal of these chemicals can lead to toxic waste generation and pollution. Ensuring that manufacturers adhere to stringent environmental regulations is crucial to minimizing the environmental impact of battery production.
Battery Degradation and Disposal:
- End-of-Life Concerns: As EV batteries reach the end of their useful life, proper disposal becomes a major concern. Without effective recycling or repurposing methods, used batteries can contribute to electronic waste (e-waste), which poses significant environmental risks. Batteries contain hazardous materials, such as heavy metals, which can leach into the soil and groundwater if not properly managed.
- Recycling Challenges: While recycling can recover valuable materials from used batteries, the process is complex and not yet fully optimized. Current recycling methods often recover only a fraction of the materials, and the processes themselves can be energy-intensive and environmentally taxing. Advancements in recycling technologies are needed to improve recovery rates and reduce the environmental impact of battery disposal.
Overall Environmental Footprint:
- Comparison with Internal Combustion Engines (ICEs): Despite the environmental challenges associated with EV batteries, studies show that electric vehicles have a lower overall environmental impact compared to internal combustion engine vehicles (ICEs) over their entire life cycle. EVs produce significantly fewer tailpipe emissions, and as the electricity grid becomes greener, the benefits of EVs will only increase. However, it is crucial to address the environmental challenges associated with battery production and disposal to fully realize the environmental benefits of EVs.
Economic Impact
Cost of Battery Production:
- Raw Material Costs: The cost of raw materials used in EV batteries has a significant impact on the overall cost of battery production. Fluctuations in the prices of lithium, cobalt, nickel, and other essential materials can affect the affordability of electric vehicles. As demand for these materials increases with the growth of the EV market, supply chain constraints, and geopolitical factors can further influence prices.
- Manufacturing Costs: The production of EV batteries involves complex manufacturing processes, which contribute to the high cost of batteries. While economies of scale and advancements in manufacturing techniques are helping to drive down costs, batteries remain one of the most expensive components of an electric vehicle. Reducing production costs is key to making EVs more affordable for consumers.
Impact on Vehicle Costs:
- Battery as a Significant Cost Component: The cost of the battery pack represents a substantial portion of the total cost of an electric vehicle, often ranging from 30% to 50%. As battery costs decrease, the overall price of EVs is expected to become more competitive with traditional internal combustion engine vehicles, making them more accessible to a broader range of consumers.
- Government Incentives and Subsidies: To encourage the adoption of electric vehicles, many governments offer incentives and subsidies to offset the higher upfront costs. These incentives often include tax credits, rebates, and grants for both consumers and manufacturers. However, the availability and magnitude of these incentives vary by region and are subject to change as the market evolves.
Job Creation and Economic Growth:
- Expansion of the EV Industry: The growth of the electric vehicle industry is creating new economic opportunities, particularly in the areas of battery manufacturing, research and development, and infrastructure deployment. As EV adoption increases, demand for skilled workers in battery production, maintenance, and recycling is expected to rise, leading to job creation in these sectors.
- Impact on Traditional Automotive Jobs: The shift from internal combustion engine vehicles to electric vehicles is likely to disrupt traditional automotive jobs, particularly in regions heavily reliant on conventional vehicle manufacturing. While new jobs are being created in the EV sector, there is a need for workforce retraining and reskilling programs to help workers transition to new roles in the evolving automotive industry.
Energy and Resource Dependency:
- Dependence on Critical Materials: The reliance on specific raw materials for battery production has implications for energy and resource security. Countries that dominate the production of lithium, cobalt, and nickel hold significant influence over the global supply chain. Diversifying sources of these materials and developing alternative battery chemistries are important strategies for reducing dependency and ensuring the stability of the EV market.
- Impact on Energy Markets: The increasing adoption of electric vehicles has the potential to reshape global energy markets. As demand for oil decreases due to reduced reliance on internal combustion engines, the focus may shift towards electricity generation and grid infrastructure. This transition could lead to changes in energy pricing, investment patterns, and the geopolitical landscape of energy production and consumption.
Long-Term Economic Benefits:
- Cost Savings for Consumers: While electric vehicles have a higher upfront cost compared to traditional vehicles, they offer long-term cost savings through lower fuel and maintenance expenses. EVs have fewer moving parts and do not require oil changes, which reduces the need for regular maintenance. Additionally, electricity is generally cheaper than gasoline, leading to lower operating costs over the life of the vehicle.
- Economic Benefits of Sustainability: The shift towards electric vehicles is part of a broader movement towards sustainability and decarbonization. By reducing greenhouse gas emissions and dependence on fossil fuels, EVs contribute to long-term economic stability and environmental resilience. The transition to a low-carbon economy can also stimulate innovation, drive investment in renewable energy, and create new economic opportunities in the green technology sector.
The environmental and economic impacts of electric vehicle batteries are complex and multifaceted. While the adoption of EVs presents significant opportunities for reducing emissions and fostering sustainable growth, it also raises challenges related to raw material extraction, manufacturing, and disposal. Addressing these challenges requires a holistic approach that includes advancements in battery technology, improvements in recycling processes, and the development of policies that support the responsible and equitable growth of the EV market.
As the electric vehicle industry continues to evolve, it is essential to balance the benefits of EVs with the need for sustainable practices and economic considerations. By doing so, we can pave the way for a future where electric vehicles are not only a viable alternative to traditional vehicles but also a cornerstone of a more sustainable and prosperous world.
Click Here to Know More About Electric Vehicle Battery Usage