Electric Vehicle Battery Capacity
Electric Vehicle Battery Capacity measures the energy storage capability of EV batteries, influencing range and performance. Higher capacity batteries enable longer trips and better efficiency, while advancements in technology continue to enhance energy density and reduce charging times. Understanding capacity is key to optimizing EV performance and user experience.
Electric Vehicle Battery Capacity
Table of Contents
- Introduction
- Overview of Electric Vehicle Batteries
- Importance of Battery Capacity in EVs
- Understanding Electric Vehicle Battery Capacity
- What is Battery Capacity?
- Measuring Battery Capacity: kWh
- Factors Affecting Battery Capacity
- Types of Batteries Used in Electric Vehicles
- Lithium-Ion Batteries
- Solid-State Batteries
- Nickel-Metal Hydride (NiMH) Batteries
- Lead-Acid Batteries
- Battery Capacity and Electric Vehicle Range
- How Battery Capacity Impacts Driving Range
- Energy Efficiency of EVs
- Real-World Factors Impacting Range
- Battery Capacity and Charging Time
- Relation Between Capacity and Charging Speed
- Fast Charging vs Slow Charging
- Charging Infrastructure
- Battery Capacity Degradation Over Time
- Causes of Battery Degradation
- How to Minimize Capacity Loss
- Battery Maintenance and Care
- Battery Capacity and Cost
- Cost vs Capacity Relationship
- High-Capacity Battery EV Models
- Future Trends in Battery Costs
- Advances in EV Battery Technology
- Increasing Energy Density
- Innovations in Battery Chemistry
- The Future of Solid-State Batteries
- Battery Capacity and Sustainability
- Recycling EV Batteries
- Environmental Impact of Larger Batteries
- Role of Renewable Energy in Charging
- Conclusion
Introduction
Overview of Electric Vehicle Batteries
Electric vehicles (EVs) have revolutionized the transportation industry by offering a sustainable alternative to internal combustion engine (ICE) vehicles. At the heart of every electric vehicle is its battery, which stores the energy required to power the vehicle’s electric motor. Unlike traditional vehicles that rely on gasoline or diesel fuel, EVs use electricity stored in high-capacity batteries to operate.
The performance, range, and overall efficiency of an electric vehicle largely depend on the type and capacity of its battery. As the electric vehicle market expands globally, there is increasing emphasis on enhancing battery technology, particularly in terms of capacity, which directly affects driving range, charging time, and long-term performance.
Importance of Battery Capacity in EVs
Battery capacity plays a critical role in determining how far an electric vehicle can travel on a single charge and how long it takes to recharge. High-capacity batteries can store more energy, allowing for extended driving ranges and less frequent charging. As EV manufacturers race to improve their vehicles’ performance, increasing battery capacity has become a focal point of innovation. This blog post explores the intricacies of electric vehicle battery capacity, its impact on EV performance, and the future of this essential component in sustainable transportation.
Understanding Electric Vehicle Battery Capacity
What is Battery Capacity?
Battery capacity refers to the total amount of electrical energy that a battery can store. It is measured in kilowatt-hours (kWh), which indicates how many kilowatts of power the battery can supply over the course of an hour. The higher the kWh rating of a battery, the more energy it can store, resulting in greater driving range for an electric vehicle.
For instance, if an electric vehicle has a 60 kWh battery, it means the battery can supply 60 kilowatts of power for one hour, or 30 kilowatts of power for two hours, and so on. This energy is then used to power the vehicle’s electric motor and other systems.
Measuring Battery Capacity: kWh
Kilowatt-hours (kWh) are the standard unit of measurement for EV battery capacity. To give context, most electric vehicles on the market today have battery capacities ranging from 20 kWh to over 100 kWh. For example:
- The Nissan Leaf, a popular EV, comes with a 40 kWh battery.
- Tesla’s Model S offers options with battery capacities of up to 100 kWh, giving it an extended driving range.
Factors Affecting Battery Capacity
Several factors can affect the usable capacity of an EV battery, including:
- Battery Type: Different battery chemistries (lithium-ion, solid-state, etc.) offer varying levels of energy density.
- Temperature: Extreme temperatures, particularly cold weather, can reduce battery performance and effective capacity.
- Age and Usage: Over time, battery cells degrade, which reduces their ability to hold a full charge.
Types of Batteries Used in Electric Vehicles

Lithium-Ion Batteries
Lithium-ion (Li-ion) batteries are the most commonly used in electric vehicles today due to their high energy density, long cycle life, and relatively low weight. Li-ion batteries are capable of storing large amounts of energy in a compact form, making them ideal for EVs.
Solid-State Batteries
Solid-state batteries are considered the next generation of EV batteries. They use a solid electrolyte instead of a liquid one, which offers higher energy density, improved safety, and faster charging times. Although not yet widely available, solid-state batteries have the potential to revolutionize the EV market.
Nickel-Metal Hydride (NiMH) Batteries
NiMH batteries were used in early hybrid electric vehicles but have largely been replaced by lithium-ion technology in modern EVs. NiMH batteries offer less energy density than Li-ion but are more durable and tolerant of extreme temperatures.
Lead-Acid Batteries
Lead-acid batteries are rarely used in modern EVs due to their low energy density and heavy weight. However, they are still used in some electric buses and trucks due to their low cost.
Battery Capacity and Electric Vehicle Range
How Battery Capacity Impacts Driving Range
The driving range of an electric vehicle is directly linked to its battery capacity. A higher-capacity battery can store more energy, enabling the vehicle to travel farther on a single charge. For example, a 100 kWh battery can provide a range of up to 370 miles (596 km) in a Tesla Model S, while a smaller 40 kWh battery in a Nissan Leaf delivers a range of around 150 miles (241 km).
Energy Efficiency of EVs
Energy efficiency plays a role in how far an EV can travel per kWh of energy stored in the battery. Vehicles with better aerodynamics, lighter weights, and efficient drivetrains can extract more miles from each kWh of battery capacity.
Real-World Factors Impacting Range
While manufacturers often advertise a vehicle’s maximum range under ideal conditions, several factors can influence the actual range in real-world usage, including:
- Driving habits (aggressive acceleration can drain the battery faster)
- Terrain (hilly areas require more energy)
- Climate control usage (heating or air conditioning consumes battery power)
- Temperature (cold weather reduces battery efficiency)
Battery Capacity and Charging Time
Relation Between Capacity and Charging Speed
The larger the battery capacity, the more energy is required to fully charge it. However, this doesn’t necessarily mean that a larger battery will take longer to charge, as charging speed depends on the power output of the charging station and the battery’s charging capacity. For example, a 100 kWh battery might take a similar amount of time to charge as a 60 kWh battery if both are charged using high-power fast chargers.
Fast Charging vs Slow Charging
Electric vehicles can be charged using different types of chargers, which vary in speed:
- Level 1 Chargers: These are standard household outlets that provide slow charging (typically adding 4-5 miles of range per hour).
- Level 2 Chargers: These provide faster charging, adding up to 25 miles of range per hour.
- DC Fast Chargers: These can add hundreds of miles of range in a short time (up to 80% charge in under 30 minutes).
Charging Infrastructure
The availability of public charging stations is key to making EVs more practical for long-distance travel. Many countries are rapidly expanding their EV charging infrastructure, which can help reduce range anxiety and allow for quicker recharging on the go.
Battery Capacity Degradation Over Time
Causes of Battery Degradation
As with all rechargeable batteries, EV batteries degrade over time. The loss of capacity is primarily due to repeated charging and discharging cycles, as well as exposure to high temperatures. Lithium-ion batteries, while generally robust, experience gradual degradation that can lead to a reduction in usable capacity and driving range.
How to Minimize Capacity Loss
There are several steps EV owners can take to minimize battery degradation:
- Avoid frequently charging the battery to 100% or letting it drop to 0%.
- Use slow chargers rather than fast chargers when possible, as fast charging generates more heat, which can degrade the battery.
- Keep the battery at moderate temperatures, avoiding exposure to extreme heat or cold.
Battery Maintenance and Care
Proper battery maintenance can extend its lifespan. Some EVs come equipped with battery management systems that help monitor the battery’s health and manage charging patterns to reduce degradation. In some cases, software updates can also help improve battery performance.
Battery Capacity and Cost
Cost vs Capacity Relationship
The relationship between battery capacity and cost is a crucial factor for both manufacturers and consumers of electric vehicles (EVs). As battery technology advances and economies of scale are achieved, the cost of battery packs has been decreasing. However, there are still significant cost implications associated with larger battery capacities.
Cost Breakdown:
- Materials: The cost of materials such as lithium, cobalt, and nickel significantly influences the overall price of the battery. Higher-capacity batteries require more of these materials, thus increasing costs.
- Manufacturing: Larger batteries are more complex to manufacture. The additional layers and components needed for higher capacity add to the production costs.
- Research and Development (R&D): Investment in R&D for advanced battery technologies and improvements in energy density and longevity contribute to the overall cost of batteries. High-capacity batteries often involve cutting-edge technologies, which can be more expensive to develop and produce.
Historically, the cost of lithium-ion batteries has been decreasing steadily. According to BloombergNEF, the cost of lithium-ion battery packs dropped from around $1,200 per kWh in 2010 to approximately $137 per kWh in 2023. This trend is expected to continue, although the rate of decline may slow as battery technologies mature.
High-Capacity Battery EV Models
Several electric vehicle models are available with high-capacity batteries, providing extended driving ranges and improved performance. These vehicles are generally more expensive but offer significant benefits in terms of range and convenience.
- Tesla Model S
- Battery Capacity: Up to 100 kWh
- Range: Over 370 miles (596 km)
- Cost: Approximately $90,000 to $110,000, depending on configuration and options.
- Lucid Air
- Battery Capacity: Up to 118 kWh
- Range: Over 500 miles (805 km)
- Cost: Starts around $80,000, with higher configurations exceeding $100,000.
- Mercedes-Benz EQS
- Battery Capacity: 108 kWh
- Range: Up to 350 miles (563 km)
- Cost: Around $105,000 to $120,000.
- Ford Mustang Mach-E
- Battery Capacity: Up to 88 kWh
- Range: Up to 300 miles (483 km)
- Cost: Ranges from $45,000 to $65,000, depending on the model and options.
- Audi e-tron GT
- Battery Capacity: 93 kWh
- Range: Approximately 238 miles (383 km)
- Cost: Around $100,000.
These high-capacity EVs are targeted at consumers who prioritize long-range travel and high performance. As battery costs continue to decrease, these models are expected to become more affordable, making long-range EVs accessible to a broader audience.
Future Trends in Battery Costs
As technology continues to evolve, several trends are likely to influence the cost of EV batteries:
- Advances in Battery Chemistry: Innovations in battery chemistry, such as the development of solid-state batteries, promise higher energy densities and potentially lower costs. Solid-state batteries could reduce the reliance on expensive materials like cobalt and nickel.
- Economies of Scale: As the adoption of electric vehicles increases, manufacturers will be able to produce batteries at larger scales, reducing per-unit costs. Increased production volumes can lead to significant cost reductions over time.
- Recycling and Reuse: Improvements in battery recycling processes can help reduce costs by recovering valuable materials from used batteries. Enhanced recycling technologies will support the sustainability of battery production and reduce overall material costs.
- Government Incentives: Policies and subsidies aimed at promoting electric vehicle adoption can indirectly affect battery costs. Government incentives for research, development, and production of EV batteries can help accelerate advancements and reduce costs.
- Technological Innovations: Emerging technologies, such as new manufacturing techniques and advanced battery management systems, can contribute to cost reductions. Innovations in energy storage and battery design will play a crucial role in shaping future battery costs.
Advances in EV Battery Technology
The electric vehicle (EV) market is rapidly evolving, and advancements in battery technology are at the forefront of this transformation. Innovations in battery design, materials, and manufacturing processes are driving improvements in performance, safety, and affordability. This section explores some of the key advancements in EV battery technology and their implications for the future of electric transportation.

Increasing Energy Density
Energy density refers to the amount of energy that can be stored in a given volume or weight of battery. The Higher energy density means that a battery can store more energy in a smaller or lighter package, which is crucial for improving the range and efficiency of electric vehicles.
- Lithium-Ion Enhancements:
- Silicon Anodes: Traditional lithium-ion batteries use graphite anodes, but researchers are increasingly exploring silicon anodes. Silicon has a higher capacity for lithium ions, which can significantly boost the energy density of batteries. Companies like Tesla and startups such as Sila Nanotechnologies are actively developing silicon-based anodes to enhance battery performance.
- High-Nickel Cathodes: Increasing the nickel content in battery cathodes can improve energy density. High-nickel cathodes reduce the amount of cobalt needed, which not only lowers costs but also addresses supply chain concerns. Companies like LG Chem and Panasonic are working on high-nickel cathode formulations to increase battery capacity.
- Solid-State Batteries:
- What They Are: Solid-state batteries replace the liquid electrolyte in traditional lithium-ion batteries with a solid electrolyte. This design enhances energy density and safety by eliminating the flammable liquid electrolyte and providing a more stable and dense energy storage solution.
- Advantages: Solid-state batteries can offer up to 50% higher energy density compared to current lithium-ion batteries. They also have a lower risk of thermal runaway and improved performance at lower temperatures.
- Current Status: While solid-state batteries are still in the development phase, several companies, including QuantumScape and Solid Power, are making significant strides. The commercialization of solid-state batteries is expected within the next few years, potentially revolutionizing the EV market.
Innovations in Battery Chemistry
Advancements in battery chemistry aim to enhance energy density, reduce costs, and improve overall performance. Key areas of innovation include:
- Lithium-Sulfur Batteries:
- Overview: Lithium-sulfur (Li-S) batteries use sulfur as the cathode material, which offers a higher theoretical energy density compared to conventional lithium-ion batteries.
- Benefits: Li-S batteries are lighter and cheaper to produce, and they have the potential to provide up to five times the energy density of current lithium-ion batteries. However, challenges such as low cycle life and poor conductivity need to be addressed before they can be widely adopted.
- Lithium-Iron-Phosphate (LiFePO4) Batteries:
- Overview: Lithium-iron-phosphate batteries are known for their safety, long cycle life, and stability. They have a lower energy density compared to some other lithium-ion chemistries but offer better thermal stability and are less prone to overheating.
- Applications: LiFePO4 batteries are commonly used in applications where safety and longevity are more critical than maximum energy density. They are increasingly being used in buses and stationary energy storage systems.
- Sodium-Ion Batteries:
- Overview: Sodium-ion batteries use sodium ions instead of lithium ions. Sodium is more abundant and cheaper than lithium, which could potentially lower battery costs.
- Current Development: While sodium-ion batteries have lower energy density compared to lithium-ion batteries, researchers are working to improve their performance. Companies like CATL are developing sodium-ion batteries for use in lower-cost EVs and energy storage applications.
The Future of Solid-State Batteries
Here’s a closer look at their potential impact:
- Enhanced Safety:
- Reduced Flammability: Solid-state batteries use a solid electrolyte instead of a liquid one, reducing the risk of fire and explosion in case of battery damage or failure.
- Thermal Stability: The solid electrolyte provides better thermal stability, making the battery safer to operate in extreme temperatures.
- Improved Performance:
- Higher Energy Density: Solid-state batteries can achieve higher energy densities, providing longer driving ranges for electric vehicles. This can address one of the major limitations of current lithium-ion batteries.
- Faster Charging: Solid-state batteries have the potential for faster charging times due to their ability to handle higher current densities without overheating.
- Commercialization Challenges:
- Manufacturing Costs: Producing solid-state batteries involves complex and costly manufacturing processes. Scaling up production to meet market demand will be a key challenge.
- Durability and Lifecycle: Ensuring that solid-state batteries maintain their performance over many charge-discharge cycles is crucial for their widespread adoption.
Role of Battery Management Systems (BMS)
Battery Management Systems (BMS) play a crucial role in optimizing the performance and safety of EV batteries. Advances in BMS technology include:
- Enhanced Monitoring:
- Real-Time Data: Modern BMS can monitor various parameters such as voltage, temperature, and state of charge in real-time, ensuring optimal battery performance and safety.
- Predictive Analytics: Advanced BMS systems use machine learning and predictive analytics to forecast battery health and performance, enabling proactive maintenance and reducing the risk of failure.
- Improved Efficiency:
- Cell Balancing: BMS technologies are designed to balance the charge across individual battery cells, preventing overcharging or deep discharging of any single cell, which helps extend battery life.
- Energy Management: BMS systems optimize energy usage and charging cycles to maximize battery efficiency and longevity.
Recycling and Sustainability
Sustainability is an important consideration in the development of EV batteries. Advances in recycling and sustainable practices are crucial for reducing the environmental impact of battery production and disposal:
- Battery Recycling:
- Closed-Loop Recycling: Innovations in recycling technology focus on recovering valuable materials such as lithium, cobalt, and nickel from used batteries. Closed-loop recycling systems aim to reuse these materials in new batteries, reducing the need for raw material extraction and minimizing waste.
- Recycling Efficiency: Advances in recycling processes improve the efficiency of material recovery and reduce the environmental footprint of battery production.
- Sustainable Materials:
- Alternative Materials: Research is underway to identify and develop more sustainable materials for battery production. This includes reducing reliance on rare or environmentally harmful materials and exploring alternative chemistries.
- Eco-Friendly Manufacturing: Efforts are being made to make battery manufacturing processes more environmentally friendly, including reducing energy consumption and minimizing emissions.
Battery Capacity and Sustainability
As electric vehicles (EVs) become more prevalent, the sustainability of battery production, usage, and disposal has become a significant focus. Battery capacity plays a critical role in this sustainability equation, influencing the environmental impact throughout the lifecycle of the battery. This section explores how battery capacity interacts with sustainability considerations, including recycling, environmental impact, and the role of renewable energy.
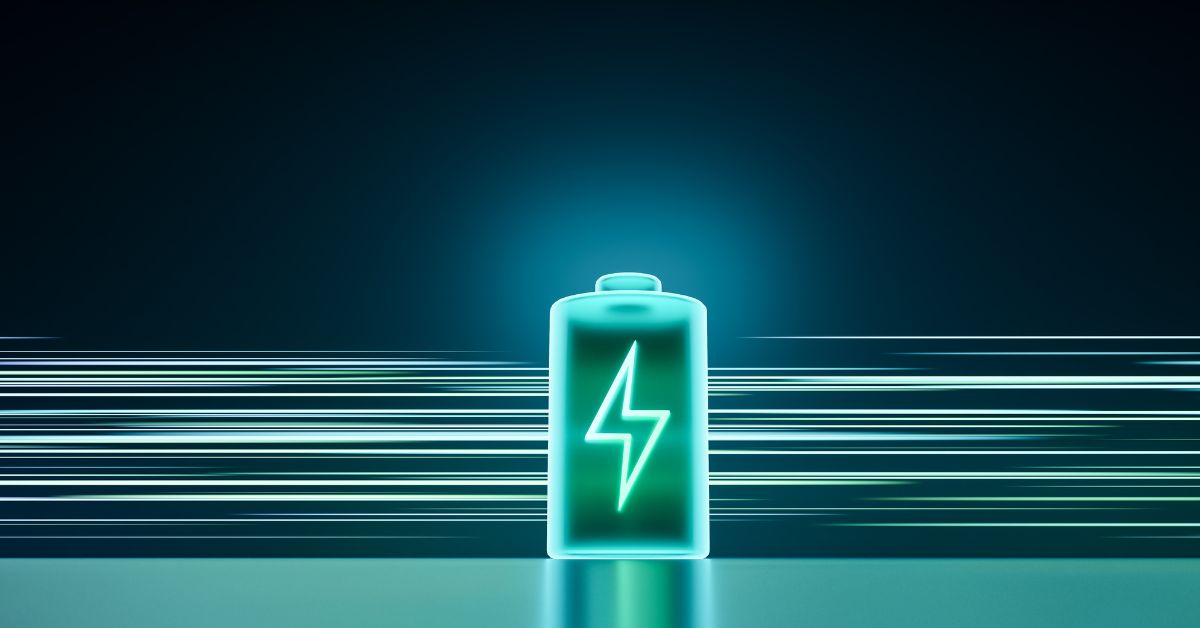
Recycling and Disposal of EV Batteries
- Battery Recycling Technologies:
- Closed-Loop Recycling: Closed-loop recycling systems aim to recover valuable materials from used batteries and reuse them in new batteries. This process reduces the need for raw material extraction and helps mitigate environmental impacts. Technologies such as hydrometallurgical and pyrometallurgical processes are used to extract lithium, cobalt, nickel, and other key materials.
- Challenges in Recycling: Despite advancements, recycling remains challenging due to the complex composition of batteries and the need to separate different materials efficiently. The industry is working to improve the efficiency of these processes and reduce the costs associated with recycling.
- Environmental Impact of Recycling:
- Resource Recovery: Effective recycling reduces the environmental impact associated with mining and processing raw materials. It also helps to lower the carbon footprint of battery production by reusing valuable resources.
- Waste Management: Proper disposal of batteries is crucial to prevent harmful substances from leaching into the environment. Regulations and standards are in place in many regions to ensure that batteries are disposed of safely and responsibly.
Environmental Impact of Battery Production
- Material Sourcing:
- Raw Materials: The production of high-capacity batteries requires substantial quantities of raw materials such as lithium, cobalt, and nickel. Mining and processing these materials can have significant environmental and social impacts, including habitat destruction, water usage, and labor concerns.
- Sustainable Sourcing: Efforts are being made to source materials more sustainably, including initiatives to reduce reliance on conflict minerals and improve the environmental practices of mining operations.
- Manufacturing Processes:
- Energy Consumption: The manufacturing of high-capacity batteries is energy-intensive. Innovations in production techniques aim to reduce energy consumption and lower the carbon footprint of battery manufacturing.
- Emission Reduction: Advances in technology and improvements in manufacturing processes are focused on reducing emissions and minimizing the environmental impact of battery production.
The Role of Renewable Energy in Charging
- Renewable Energy Integration:
- Green Charging: Integrating renewable energy sources such as solar, wind, and hydro into EV charging infrastructure can significantly reduce the carbon footprint associated with charging electric vehicles. Charging stations powered by renewable energy contribute to the overall sustainability of EVs.
- Solar-Powered Charging Stations: Some charging stations are equipped with solar panels to generate clean energy on-site. This approach not only reduces reliance on grid power but also promotes the use of renewable energy.
- Impact on Battery Life:
- Optimal Charging Conditions: Using renewable energy for charging can help maintain optimal charging conditions, which can positively impact battery health and longevity. Consistent, low-carbon charging reduces the likelihood of overheating and degradation.
- Energy Storage Solutions: Integrating battery storage solutions with renewable energy systems can help manage the intermittency of renewable sources and provide a more stable and sustainable energy supply for EV charging.
Sustainability of High-Capacity Batteries
- Energy Density vs. Sustainability:
- Trade-Offs: High-capacity batteries offer extended driving ranges and improved performance, but they also require more materials and energy to produce. Balancing energy density with sustainability involves optimizing the use of resources and minimizing environmental impacts.
- Material Efficiency: Advances in battery chemistry and design aim to increase energy density while reducing the amount of raw materials required. Innovations such as solid-state batteries and new electrode materials are expected to improve efficiency and sustainability.
- Lifecycle Assessments:
- Holistic View: Lifecycle assessments (LCAs) evaluate the environmental impact of batteries from production through to disposal. These assessments help identify areas for improvement and guide efforts to enhance the sustainability of battery technologies.
- Continuous Improvement: Ongoing research and development focus on improving the overall sustainability of battery systems. This includes developing new materials, optimizing production processes, and enhancing recycling technologies.
Future Directions in Battery Sustainability
- Research and Development:
- New Materials: Research is focused on finding alternative materials that are more abundant and less environmentally damaging than current battery materials. This includes exploring options like sodium-ion and magnesium-ion batteries.
- Enhanced Recycling Methods: Innovations in recycling technologies aim to improve the efficiency and effectiveness of material recovery, making battery recycling more viable and cost-effective.
- Policy and Regulation:
- Supportive Legislation: Governments and regulatory bodies play a crucial role in promoting battery sustainability through policies and regulations. This includes setting standards for recycling, encouraging the use of renewable energy, and supporting research into sustainable battery technologies.
- Incentives for Green Practices: Incentives and subsidies for adopting green technologies and practices can drive the adoption of sustainable battery solutions and accelerate progress in battery sustainability.
Conclusion: Electric Vehicle Battery Capacity
Electric vehicle (EV) battery capacity is a fundamental aspect of the electric transportation revolution, influencing everything from vehicle performance to environmental impact. As the industry continues to evolve, understanding the implications of battery capacity on range, cost, and sustainability is essential for both consumers and manufacturers.
Click Here to Learn More About Electric Vehicle Battery Capacity
Click Here to Learn More About Electric Vehicle Battery Price